At the heart of any biological process, from DNA replication and enzymatic activity to cellular motion and transformations, are small mechanical cues and forces that help drive reactions and guide synthetic pathways. As research pushes the frontiers of medicine and bioengineering, tracking and quantifying molecular-level forces, displacements, and torques will be become a critical component to unraveling the mysteries of the cell and Nature’s marvelous ability to sustain life. Furthermore, measuring nanomechanical events or mechanical responses is essential for developing novel label-free diagnostic devices and analytical probes. Most molecular machines and biological systems operate in the piconewton range and in complex media which puts immediate constraints on instrumentation. Of the many nanomechanical detection platforms, atomic force microscopes (AFMs) and optical/magnetic traps have emerged as the work-horse instruments that can resolve and extract quantitative force information from biological systems. However, it remains difficult to access the intracellular environment with these analytical tools, or multiplex the force read-outs, as they have fairly large sizes and feedback mechanisms. Our goal is to develop a new breed of nanofiber optic devices that not only can measure ultrasmall displacements (Å level) and forces (< 1 pN) but have the capacity to be multiplexed far beyond current state-of-the-art nanomechanical instruments.
(left) Schematic of the fiber optic force sensor along with an SEM image of a Au nanoparticle on a SnO2 nanofiber waveguide. (right) Intensity time-course of a single Au nanoparticle scattering in the near-field of a nanofiber waveguide. The nanoparticle is tethered to the waveguide via a single DNA strand and shows 1 Å distance sensitivity while the DNA is compressed or stretched under fluid flow.
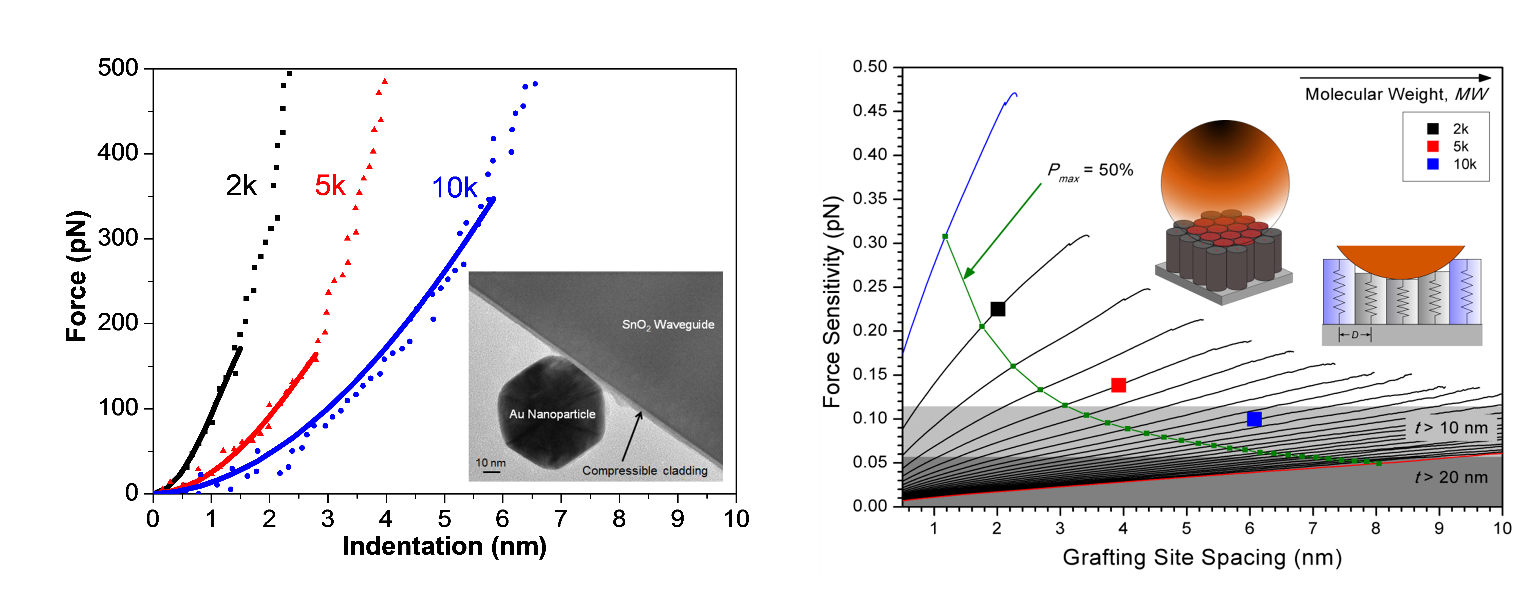
(left) Nanoindentation curves of different molecular weight PEG coatings on a nanofiber waveguide. The TEM micrograph captures a nanofiber optic force transducer. (right) Modeled force sensitivity of the nanofiber optic force transducer for various PEG molecular weights as a function of inter-chain spacing.
To do this we have been investigating near-field light-matter interactions occurring within evanescent field of SnO2 subwavelength optical waveguides. For example, when a plasmonic nanoparticle is embedded in the evanescent field of the waveguide, the particle behaves like an optical antenna and expels photons from the core. By tracking the optical signal in the far-field, distance-dependent optical signals can be tracked with high resolution. Compared to fluorescent nanoparticles, we have shown that the plasmonic nanoparticles display over an order of magnitude improvement in the distance sensitivity reaching a resolution of 1 Å which is comparable to the best optical traps. To convert this molecular ruler platform into a force transducer there must be a mechanical resistance placed in between the nanoparticle and the waveguide. This can come in the form of thin, compressible fiber claddings such as polyethylene glycol (PEG) or polyelectrolyte multilayers. We now have well established protocols for synthesizing these polymer coatings and characterizing their mechanical properties via AFM. By tuning the thickness and stiffness of these films, the sensitivity and dynamic range of the transducers can be programmed. In addition to the experimental work, we have developed mechanistic models to predict the performance of these nanomechanical transducers and help guide the synthetic efforts. Ongoing work now includes experimentally calibrating the performance of these transducers and applying them to intracellular and biomolecular studies.
Related references
1. D.J. Sirbuly, M. Law, J.C. Johnson, J. Goldberger, R.J. Saykally, and P.D. Yang “Nanoribbon waveguides for subwavelength photonics integration” Science 305, 1269-1273 (2004).
2. D.J. Sirbuly, M. Law, P. Pauzauskie, H.Q. Yan, A.V. Maslov, K. Knutsen, C.Z. Ning, R.J. Saykally, and P.D. Yang “Optical routing and sensing with nanowire assemblies” Proc. Natl. Acad. Sci. U.S.A. 102, 7800-7805 (2005).
3. D.J. Sirbuly, N.O. Fischer, S.C. J. Huang, A.B. Artyukhin, J.B.H. Tok, O. Bakajin and A. Noy “Biofunctional subwavelength optical waveguides for biodetection” ACS Nano 2, 255-262 (2008).
4. I. Yoon, K. Kim, S. E. Baker, D. Heineck, S. C. Esener and D. J. Sirbuly “Stimulus-responsive light coupling and modulation with nanofiber waveguide junctions” Nano Lett. 12, 1905-1911 (2012).
5. I. Yoon, S. E. Baker, K. Kim, Y. Wang, S. C. Esener and D. J. Sirbuly ” Profiling the evanescent field of nanofiber waveguides using self-assembled polymer coatings” Nanoscale 5, 552-555 (2013).
6. I. Yoon, S. E. Baker, K. Kim, N. O. Fischer, D. Heineck, Y. Wang, S. C. Esener and D. J. Sirbuly “Nanofiber near-field light-matter interactions for enhanced detection of molecular level displacements and dynamics” Nano Lett. 13, 1440-1445 (2013).
7. Q. Huang, I. Yoon, J. Villanueva, K. Kim and D. J. Sirbuly “Quantitative mechanical analysis of thin compressible polymer monolayers on oxide surfaces” Soft Matter 10, 8001-8010 (2014).
8. J. Villanueva, Q. Huang and D. J. Sirbuly “Identification and design of novel polymeric nanomechanical transducers: A nano-structural model for thin film indentation” J. Appl. Phys.116, 104307 (2014).